Over the past few decades, limb-preserving surgery has become the best option for treating malignant bone tumours due to advances in neoadjuvant chemotherapy, imaging and surgery. Traditional oncologic surgery may present risks of local recurrence, prosthesis loosening and infection due to incomplete tumour removal or inappropriate prosthesis matching. With the application of 3D printing technology in orthopaedic surgery, it has revolutionised the traditional concept of bone tumour surgery, with its accuracy, stability and safety features making precise tumour resection and personalised reconstruction possible.
3D printing technology, also known as additive manufacturing, is a type of additive manufacturing technology, which is based on a pre-designed shape and is manufactured by continuous layer-by-layer stacking. A growing number of 3D printed products are appearing in various industries and 3D printing technology has been widely used in several clinical disciplines with great success in practice. Commonly used additive manufacturing techniques in orthopaedics include stereolithography, selective laser sintering and fused deposition moulding. Due to the complex anatomy of bone tumours, which often involve important joints, precise tumour removal is required during surgery to avoid damaging critical nerves and blood vessels, and the irregular shape of the defect after tumour removal poses a great challenge for its reconstruction. At present, 3D printing technology has been used throughout the surgical treatment of bone tumours, and combined with the assistance of various digital orthopaedic technologies, it provides patients with precise treatment solutions. This article reviews the progress of the application of 3D printing technology in bone tumour surgery.
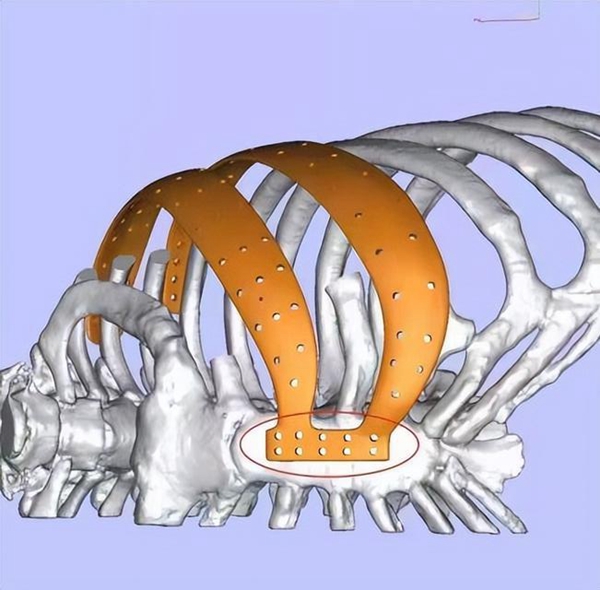
Source: Wuhan Union Medical College Hospital
Overview of digital orthopaedic technology
Digital orthopaedics is an emerging interdisciplinary discipline that integrates computer digital technology with orthopaedic treatment, thus enabling precise surgical treatment and providing better options for surgeons and patients. Currently, digital orthopaedic techniques commonly used in the surgical treatment of bone tumours include 3D printing, image fusion, computer navigation and finite element analysis, which address many of the challenges associated with traditional bone tumour surgery. It is noteworthy that 3D printing technology has achieved satisfactory efficacy in the clinical treatment of bone tumours, but there are still some problems that need to be improved. Therefore, in recent years, many scholars have proposed a precision surgical treatment strategy with 3D printing technology as the core and other digital orthopaedic technologies jointly applied as an aid, which can more scientifically assess the patient's condition and formulate the best surgical strategy, thus achieving more precise tumour resection and defect reconstruction, bringing benefits to patients (see Figure 1).
Application of 3D printing technology in preoperative planning of bone tumours
In bone tumour surgery, 3D printed anatomical models are the easiest way to assist in tumour resection. 3D printed models are obtained preoperatively from the patient's CT and MRI data to identify the anatomical structure of the target area, and then converted into digital 3D models using specialist software to segment and process them. Compared with 2D imaging images, 3D printed models can reflect the boundaries and surrounding anatomical structures of bone tumours in a more three-dimensional and intuitive way, enabling surgeons to sense the true size of the tumour, make more accurate diagnosis and surgical planning, better protect important blood vessels and nerves during surgery, and avoid post-operative complications. Kong Jinhai et al. reported the clinical application of 3D printed models in the resection of chordoma. The 3D printed models created preoperatively from thin layer CT data successfully demonstrated the lesion site and anatomy, allowing the surgeon to visualise the relationship between the tumour boundary and normal tissues, and successfully guided the surgeon in the development of the preoperative surgical plan.
Although 3D models can be successfully reconstructed with CT data to identify lesion sites, CT data cannot accurately distinguish soft tissue structures, and there is still some error with the actual tumour intraoperatively. Therefore, some scholars have proposed to fuse CT data with MRI for modelling, taking advantage of their respective advantages to clearly present soft tissues and hard tissues such as bones, so as to make tumour resection in special areas more realistic and improve surgical efficiency and safety. Zhang Ya et al. used 3D MRI sequences combined with CT modelling alignment to completely reproduce the anatomy of sacral tumours using the advantages of natural imaging of soft tissues by MRI. The sacrum is adjacent to the pelvic organs and has complex nerve and vascular accompaniment. The 3D printed model distinguishes different structures by different colours, which gives the operator a deeper understanding of the spatial relationship between the tumour and the nerves and blood vessels, effectively assisting the surgeon in preoperative planning and simulation, improving the accuracy of resection and reconstruction, shortening the operative time and reducing complications on the basis of ensuring surgical efficacy. Cherkasskiy et al. used fused deposition manufacturing (FDM) combined with ABS resin fibres to produce a 3D printed model of the proximal femur, which was used for planning and clinical application of proximal femoral osteotomy by setting the printing parameters of the honeycomb filling pattern to simulate the resistance of real bones during osteotomy and achieved good surgical results. This model has transformed from likeness to likeness, providing surgeons with new ideas to solve the problem of preoperative simulation of complex surgery. In addition, the Centre has used 3D printed tumour models in clinical teaching for medical students to gain a more intuitive and in-depth understanding of the steps and anatomical structures associated with bone tumour surgery, which has wide application prospects in clinical teaching. With the advancement of 3D printing technology and image fusion technology, 3D printed models break through the limitations of traditional technology and offer great potential for preoperative planning. The author believes that materials and printing technology that can simulate the texture of bone tissue will be the next research direction.
3D printing technology aids intraoperative precision treatment of bone tumours
The application of 3D printed guides for precise tumour resection The key to successful bone tumour surgery is to preserve as much normal bone tissue as possible, as well as important tendons and ligaments, while precisely removing the tumour to ensure the patient's functional recovery after surgery. 3D printed personalised guides allow the operator to perform more accurate preoperative planning and achieve precise intraoperative osteotomy, changing the paradigm of traditional surgery. After the preoperative 3D reconstruction of the lesion area using the patient's imaging data, the resection and reconstruction of the tumour is simulated by computer so as to determine the extent and boundaries of the tumour resection. During tumour resection, an osteotomy guide can be designed to fit the tumour site bones, and the area to be osteotomised can be designed as a groove-like structure to allow for precise intraoperative positioning of the osteotomy.
In a previous study, the advantages and disadvantages of different printing techniques and materials for intraoperative application of the guides were evaluated and compared with a control group, and it was found that the use of 3D printed guides significantly shortened the operative time, reduced the number of intraoperative fluoroscopies, and resulted in complete resection of the tumour with good reconstructive outcomes on postoperative imaging. The results showed that the use of the guide significantly reduced intraoperative bleeding and osteotomy length, reduced the risk of complications, achieved precise resection of long segmental bone defects, and improved the stability of the knee reconstruction, effectively improving the functional recovery of the knee after surgery. The 3D-printed guides were used to assist in the resection of tumours with good clinical efficacy, but the increased complexity of the osteotomy plane led to a decrease in the accuracy of intraoperative positioning of the guides and increased osteotomy errors, which posed a challenge for reconstruction. Therefore, Wong et al. proposed to combine computer-aided navigation technology with 3D-printed guides to provide a new idea for joint-preserving bone tumour surgery. The intraoperative computer navigation can provide real-time image feedback to the surgeon and verify the position of the guides, accurately guiding the tumour resection and achieving precise resection of joint osteosarcoma with excellent postoperative functional recovery. The combined use of navigation technology and 3D printed guides for the reconstruction and positioning of the acetabulum after pelvic tumour resection was found to avoid both the complex procedures associated with navigation technology alone and the errors associated with the use of the guides alone, with the postoperative assessment showing good acetabular position and reconstruction results closer to physiological reconstruction and satisfactory functional recovery. The 3D printed guide can significantly improve the surgical efficiency and reduce the incidence of complications. Combined with computer-assisted navigation technology, it can further improve the accuracy of tumour removal and achieve good postoperative reconstruction results.
For patients with bone tumours, especially malignant ones, the reconstruction of bone defects after tumour resection has always been a challenge for surgeons, with traditional reconstruction methods having their own limitations. The defect is designed and printed to match the morphology of the bone defect and have the appropriate mechanical strength to reconstruct the bone defect after tumour resection, while the overall design of the material in terms of strength, surface morphology and weight is carried out. Before the design is finalised, finite element analysis-assisted modelling can be used to test whether the prosthetic structure can withstand physiological loading and optimise the design to minimise irritation, stress concentration and stress shielding of the overlying soft tissues, while reducing the weight of the prosthesis and aiding the growth-in of the surrounding soft tissues to restore normal anatomy and physiology. To achieve immediate and long-term stability, metal 3D printing allows the production of implants with complex shapes and internal porous structures that can be controlled to the micron level, and the porous structure promotes osseointegration of the prosthesis with the remaining bone contact surfaces, facilitating early bone ingrowth and allowing the prosthesis to have good mechanical-biological properties.
Zhao et al. reported the application of 3D printed personalised prosthesis in the reconstruction of large segmental bone defects after malignant tumour resection. The study found that osseointegration occurred at the prosthesis-bone interface in all patients, and the porous structure of the prosthesis provided early biostability for the patients, who all achieved satisfactory limb function. At the same time, the Centre has developed a 3D printed prosthesis composite with vascularised fibula and bioceramic technology. The introduction of bioactive implants provides sufficient blood flow within the prosthesis to better achieve early osseointegration in patients, reducing complications and enabling the biological reconstruction of long-segment weight-bearing bone defects in the lower limb. In addition, to address the difficulty of reconstructing irregular bone defects after bone tumour resection, our centre has applied 3D printed personalised prostheses to the reconstruction of the clavicle, scapula and iliac bone for the first time, meeting the precise matching of the morphology and size of the patients' bone defects and providing a new option for limb preservation surgery in specific areas. The efficacy of the 3D printing group achieved more precise tumour resection and prosthesis matching, reduced surgical trauma, shortened operative time and facilitated postoperative functional recovery of patients compared to traditional reconstruction methods.
Despite the satisfactory results of 3D printed prostheses in clinical practice, the commonly used 3D printed implants in clinical practice are mainly titanium and its alloys. Titanium prostheses have problems such as heavy weight, poor marginal soft tissue cutting and osteoinduction, which affect their further use in clinical practice, and therefore it is particularly important to develop more suitable 3D printed materials. Our centre has tried 3D printing PEEK shoulder prostheses for clinical use. The PEEK material has a short preparation cycle, is lightweight and has good soft tissue biocompatibility, enabling good post-operative function and shape recovery. This problem is likely to be solved as materials and preparation techniques improve. For the first time, our centre has developed a 3D printed individualised tantalum metal prosthesis and used it for the reconstruction of defects after the removal of whole spinal malignant tumours. In conclusion, the author believes that the application of personalised prosthesis can achieve precise reconstruction of patients' bone defects, promote the integration of early prosthesis-bone interface and is more conducive to patients' functional recovery.
3D bioprinted bone tissue engineering scaffolds in the surgical treatment of bone tumours
Malignant bone tumours face the risk of local tumour recurrence and metastasis after surgery. Although 3D printing technology currently allows for more precise treatment in bone tumour surgery, it is still difficult to completely remove residual tumour cells through surgical intervention alone, and post-operative radiotherapy treatment is often required. However, post-operative chemotherapy and radiotherapy can lead to serious toxic side effects, and radiotherapy can cause depressed immunity, infection and inadequate blood supply to patients, which can affect the healing of bone defects. Therefore, it is important to develop new biomaterials with dual functions of residual cancer cell removal and bone defect repair. 3D printed tissue engineering scaffolds with precise layer-by-layer fabrication to assemble scaffolds, growth factors and cells can accommodate bone defects of different shapes and sizes according to pre-design, while their large interconnected pores provide sufficient space for cellular activity, nutrient transport and drug delivery This has become a hot topic of interest for researchers.
Long et al. designed a novel multifunctional PLGA/Mg composite scaffold for the postoperative treatment of osteosarcoma, in which Mg particles successfully removed residual cancer cells through photothermal effects and modulation of the tumour microenvironment under the action of near-infrared light, and achieved excellent bone defect repair by combining the unique advantages of Mg and bionic porous structure in osteogenesis. Liu et al. reported the study of element-doped bioglass for bone tumour treatment. The bioactive elements such as Cu, Fe, Mn and Co had excellent photothermal effect and osteogenic differentiation ability, and the functional scaffolds induced by the bioactive elements had better biosafety and compatibility compared with conventional nano-photothermal agents, and significantly inhibited tumour growth in vivo. Dang et al. developed a multifunctional drug-release scaffold in which adriamycin, paclitaxel and cefazolin as model drugs were successfully loaded into a 3D printed MPCL porous scaffold by immersion. This novel scaffold can be simply loaded with specific doses of drugs during surgery to prevent tumour recurrence or local infection.
In addition, our Centre has designed a new multifunctional magnesium-coated titanium scaffold that not only has osteogenic and pro-angiogenic effects, but also inhibits the growth of osteosarcoma cells through the release of Mg ions, contributing to further clinical translation of magnesium-coated titanium prosthesis. At present, a large number of studies have been reported on tissue engineering scaffolds for bone defect repair, but the development and application of 3D printing technology for bone tumour treatment is still in its infancy, and there is still a need to continuously explore and find the most suitable method to address the real bone tumour treatment.
Summary and outlook
The emergence of 3D printing technology has changed the traditional surgical approach throughout bone tumour surgery, combining the application of various digital orthopaedic technologies such as image fusion technology, computer-aided navigation and finite element analysis to successfully achieve precise treatment for patients. However, the clinical application of 3D printing technology is only in recent years and still lacks sufficient clinical sample size to illustrate the problem compared to traditional techniques, while facing problems such as high printing costs, long manufacturing cycles, lack of precision and poor tissue mimicry. At present, research on clinically applied 3D printed implants is focused on how to achieve better